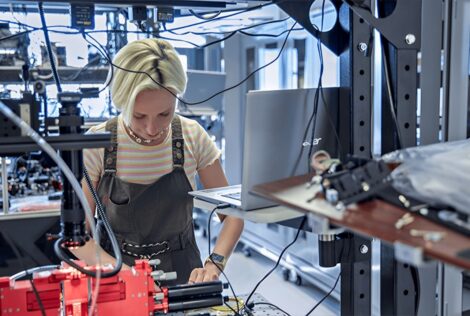
Expertise
Solar cells (photovoltaics), photodetectors, quantum information processing (quantum computers)
Areas of Specialization
Research Clusters
Current status
-
Accepting graduate students
-
Professor
Engineering Physics
Overview
Currently accepting graduate students
Research
Our current work focuses on the growth and characterization of semiconductor materials, and their application in solar cells (photovoltaics), photodetectors, infrared cameras, light sources, sensors, quantum information processing, and other optoelectronic devices. We employ a semiconductor deposition technique (molecular beam epitaxy) for growth of III-V semiconductor alloys containing In, Ga, Al, As, P, Sb and N (such as GaAs, InP, InSb, InAs, GaP, etc.). A wide range of deposition, processing techniques and devices are under investigation. Recently, our focus has been on the growth, characterization, and device applications of semiconductor nanowires. We have a large effort to develop third generation solar cells and detectors using nanostructures.
The central challenge in photovoltaic (solar cell) design is the cost-effective conversion of sunlight to electricity over the very broad range of wavelengths present in the solar spectrum. Currently, the vast majority of the photovoltaic (PV) market is made up of silicon (Si) flat panel solar cell modules. This is a relatively mature technology, with commercial flat panels largely manufactured from bulk single-crystal or multi-crystalline Si wafers with typical conversion efficiencies approaching or even exceeding 20%, and approaching the theoretical efficiency limit of 30%. There is therefore a need for innovative thinking to push Si solar cell technology beyond its current limitations. The highest solar cell efficiencies to date have been achieved using thin films of III-V semiconductor materials stacked together in tandem sub-cells connected electrically in series. Power conversion efficiencies of III-V cells are the highest of any technology, reaching over 30% in flat panels and exceeding 44% under concentrated sunlight. However, such devices cannot be grown on Si due to lattice-mismatch, and are generally grown instead on GaAs or Ge substrates that are prohibitively expensive. As a result, the application of III-V materials in terrestrial PV has been very limited. To overcome these limitations, it is highly desirable to combine the high performance of III-V materials with the relatively low cost and mature Si technology, thereby achieving the best of both worlds. To achieve this goal, recent work in photovoltaics is beginning to exploit intentionally engineered III-V semiconductor nanowire arrays that can be grown directly on Si. Our work has shown that a III-V nanowire array integrated with Si is capable of 34% PV efficiency (1 Sun) or 42% under concentrated light (500 Suns). Despite these advantages, the best reported efficiency for III–V nanowire arrays is much lower than their expected efficiency. The reasons for such modest performance are presently unclear, and further research in this field is needed. We are investigating all aspects of III-V nanowire growth on Si to produce a high efficiency, low cost solar cell technology.
We are also developing III-V nanowires monolithically integrated with Si and their potential in wavelength tunable infrared cameras. III-V nanowires support optical resonant modes such that nanowires act as very effective waveguides that concentrate and absorb light over a length of only a few microns, enabling very efficient photodetectors and solar cells with relatively little material. It is also of great interest to develop multi-spectral or wavelength discriminating infrared detectors or cameras for advanced infrared imaging systems. The resonant absorption in nanowires shows wavelength selectivity that can be tuned continuously across the visible and infrared wavelengths by adjusting the nanowire diameter. This principle can be used as a new concept for multi-spectral imaging. Lattice-mismatched III-V nanowires can be grown directly on Si substrates enabling integration with existing Si CCD or CMOS sensors. These capabilities enable large-area, low cost infrared sensors with multi-spectral capability integrated into existing Si technology.
Ph.D., Engineering Physics, McMaster University
M.Eng., Engineering Physics, McMaster University
B.Sc., Physics, Dalhousie University
Please refer to Google Scholar or Research Gate for all publications.